Qixin (Iverson) Yu
ARCHITECTURE AS (A) CARBON (-BASED) PRACTICE
Carbon serves as both a silent protagonist and a looming antagonist in the narrative of architecture, shaping not only the physical structures we inhabit but also the ecological legacy we leave behind.Centuries of human exploitation of the environment have led to climate and material crises. Shifting this dynamic requires action at micro (matter), meso (material), and macro (materiality) levels. Biogenic materials offer significant potential for carbon sequestration and present opportunities for the building industry to collaborate with nature rather than merely extract from it.
Demonstrating through Central Falls and Blackstone River, this thesis establishes a research and manufacturing practice that prioritizes material innovations, carbon sequestration, environmental rehabilitation, and adaptive reuse. By strategically sourcing local materials such as water chestnut, sawdust, and automobile tires, this thesis transforms these waste products or carbon-sequestering substances into viable building materials through scientific experimentation and testing, ultimately integrating them into architectural systems.
Industrialization, manufacturing, and construction require carbon concentration. The Blackstone River, extending 46 miles from Massachusetts to Rhode Island, was the cradle of American industrialization. Its dammed waters powered over 1,000 textile and woodworking mills, fueling economic growth and urban expansion. However, this rapid industrialization led to significant environmental degradation, resulting in hundreds of tons of trash, metal, construction waste, automobile parts, and tires in the river and its connected waterways. The restoration effort initiated by “Operation ZAP” in 1972 continues today. Water chestnut, an invasive aquatic plant introduced from Asia, has proliferated in the Blackstone River, displacing native species and offering minimal ecological value. Its polluted habitat precludes its use for food or fertilizer, further contributing to significant landfill waste.
Image
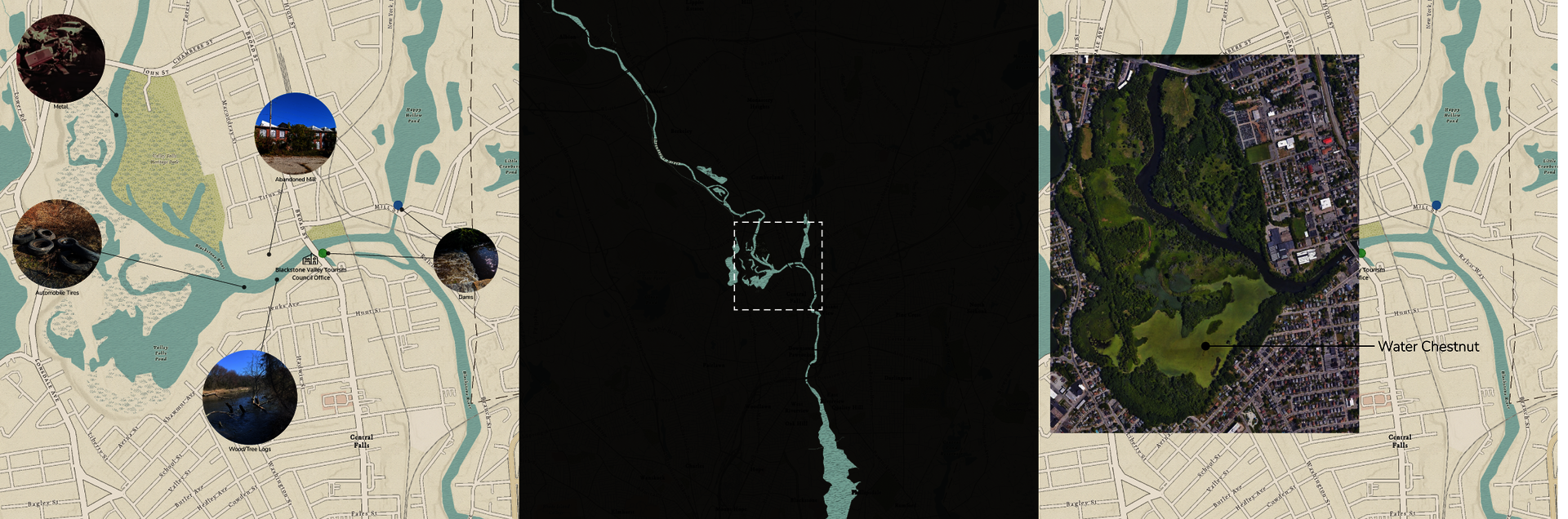
Site: Central Falls and Blackstone River
By exploring biogenic and waste materials that can be found on site, a series of experiments and tests were conducted in the RISD Nature Lab and Brown Design Workshop to develop new plant-based materials for use as viable building materials. A similar fabrication procedure is applied to both sawdust and water chestnut, involving grinding, mixing with agar-based polymer binders, and ultimately press-forming into various shapes or 3D printing as part of an additive manufacturing process.
Image
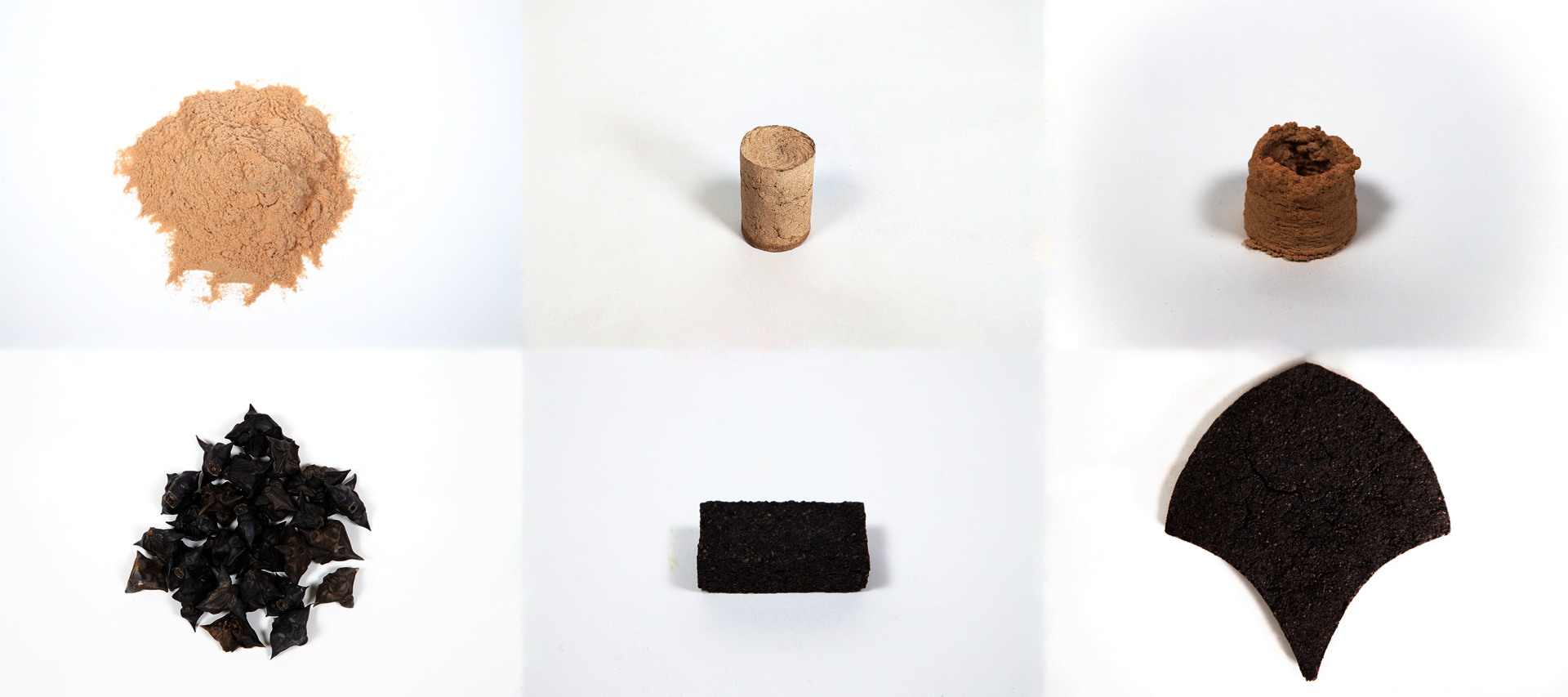
Matter, Material, Materiality: Transformation of Sawdust and Water Chestnut
The sawdust was finely ground to increase material density. Examining on the micro scale, sawdust granules are interlocked and bonded with an agar-based binder.
Image
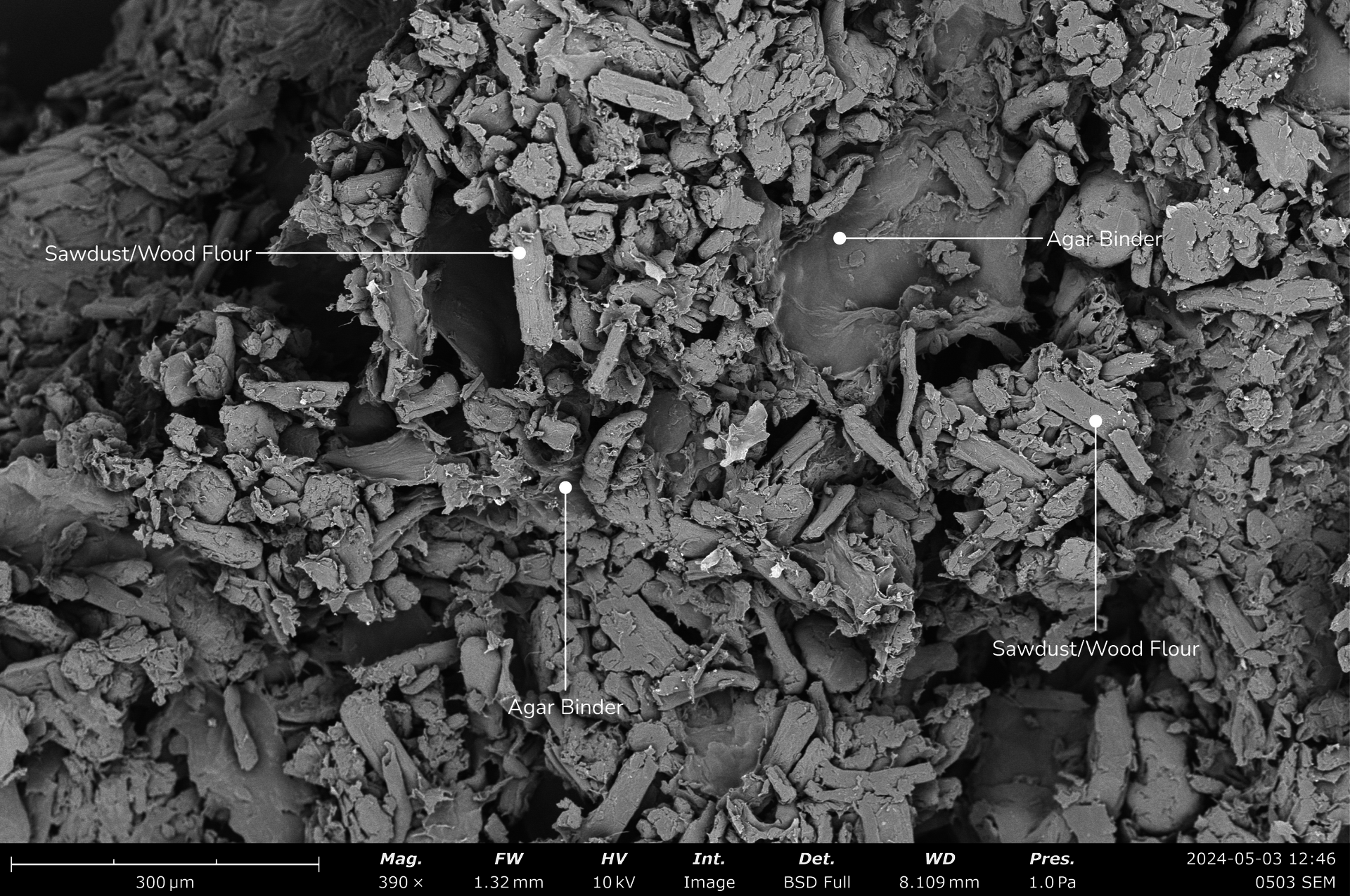
SEM Image of Sawdust Mix
Sawdust is obtainable at every stage of the natural wood cycle: from in-forest harvesting to in-house milling and sawing, and during furniture and building element manufacturing. It can constitute up to 70% of a wood-working facility's total net weight output. Once ground and mixed with polymer binders like agar and/or MCC (microcrystalline cellulose), sawdust can form a paste-like texture. This mixture can then be shaped into various molds or, with appropriate viscosity, 3D printed from a paste extruder to accommodate more intricate shapes or engineered into panelized systems. At the end of its lifespan, products and building elements made from sawdust can be broken down into powders again and reintroduced into the same manufacturing streamline or used as mulch to enrich forest soil. Alternatively, they can be utilized as biomass fuel. Although this releases locked carbon back into the atmosphere, the energy produced can help offset some carbon emissions
Image
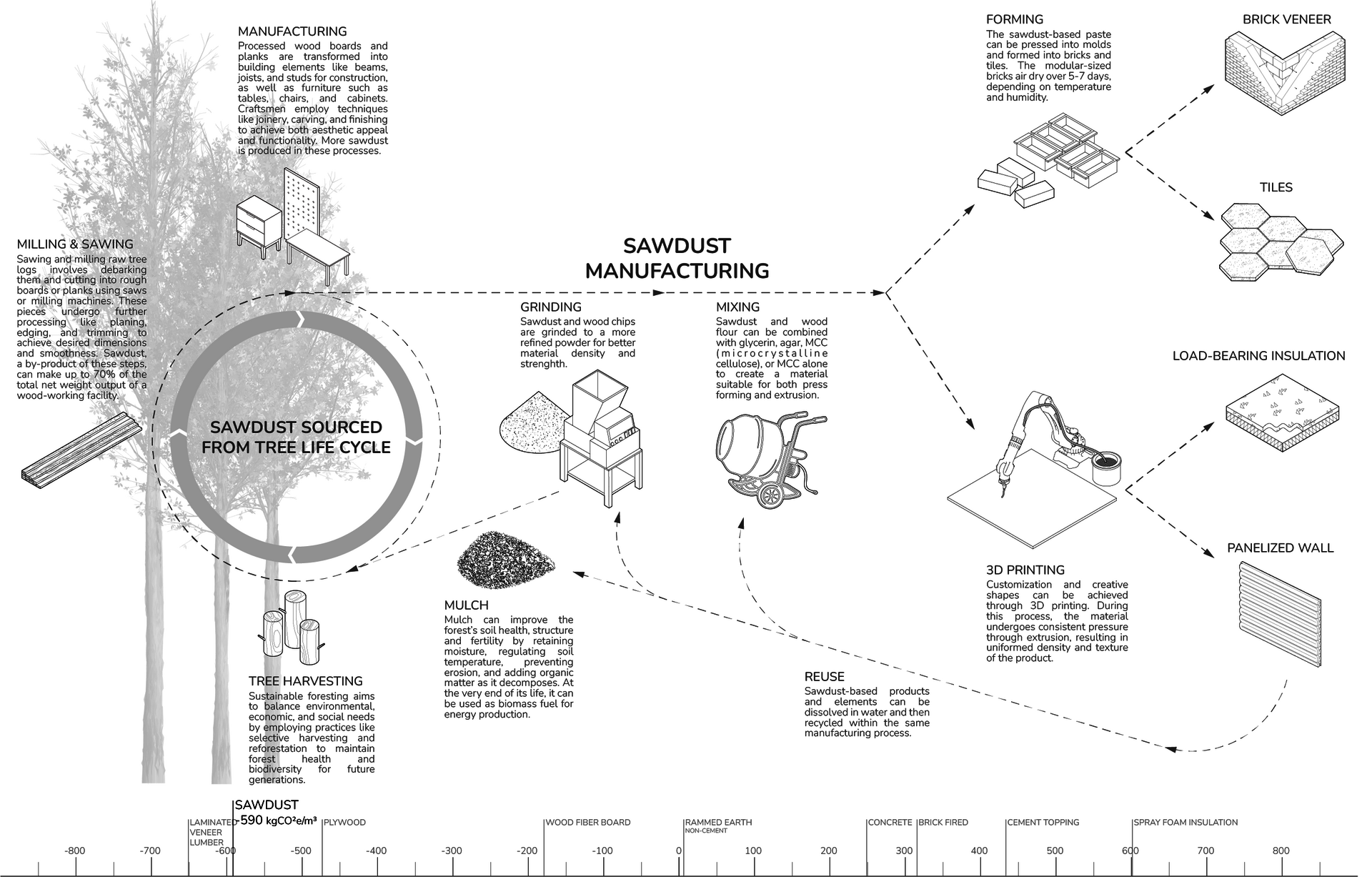
Life Cycle: Sawdust Manufacturing
Water chestnut granules are much larger than sawdust, providing more space for the agar binder to fill when mixed. As water evaporates, the sample becomes lighter in weight and more permeable.
Image
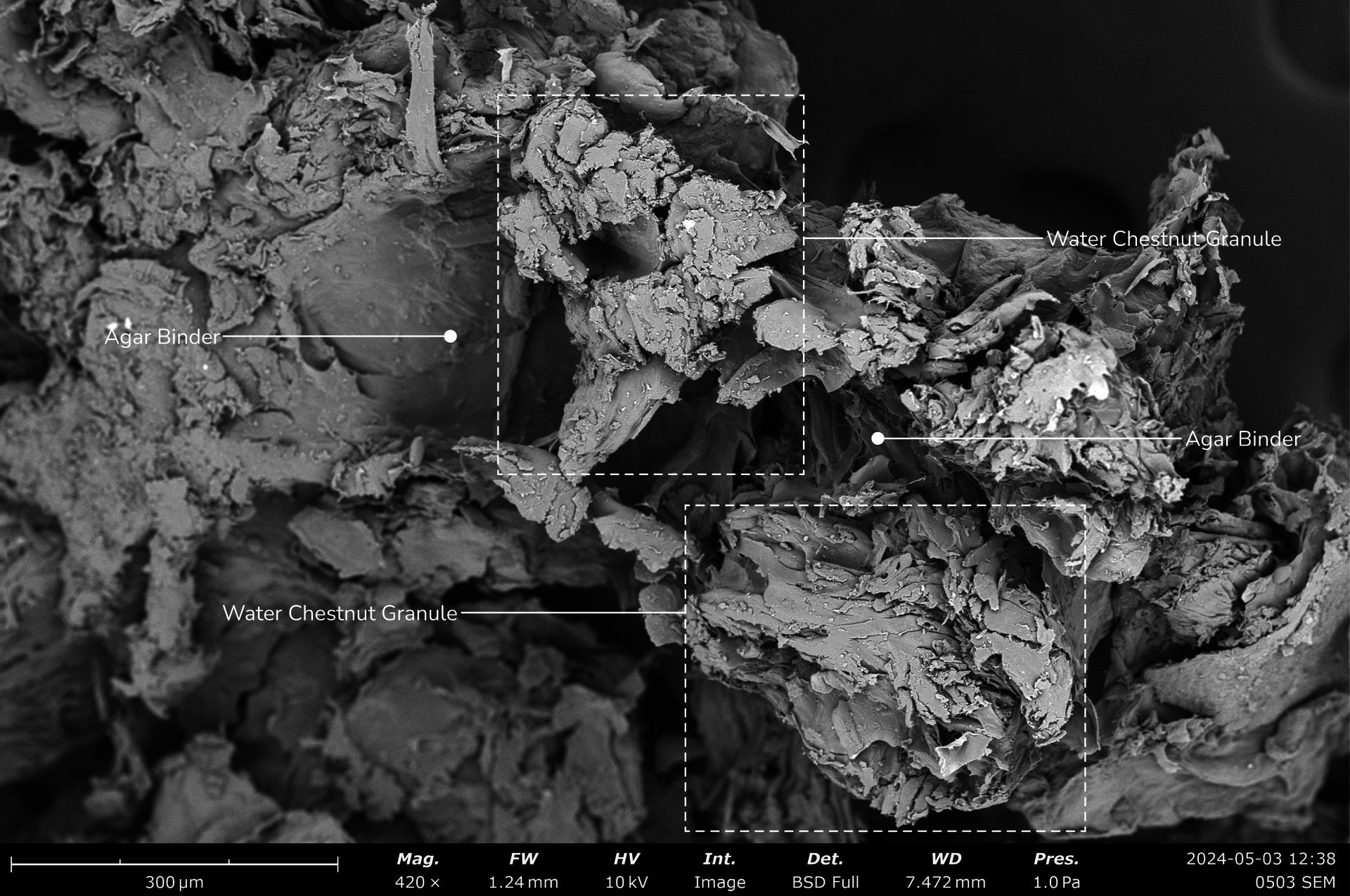
SEM Image of Water Chestnut Mix
As an annual plant, water chestnuts sprout in early spring and can be harvested in late summer and fall. Due to their rapid reproduction rate, they quickly cover water surfaces with thick floating leaves, depriving sunlight from reaching the riverbed and suffocating native plants and animals. The harvesting method aligns with the RI Environment Management Department's population control recommendation, involving pulling, cutting, and drying the plants to kill seeds and separate fibers from the meat.
Afterward, water chestnut shells undergo a similar manufacturing process involving grinding and mixing with bio-based binders. Depending on density, the resulting paste-like material can be molded or used for 3D printing. Final products range from tiles to bricks and insulation, with end-of-life options of reuse or composting.
Image
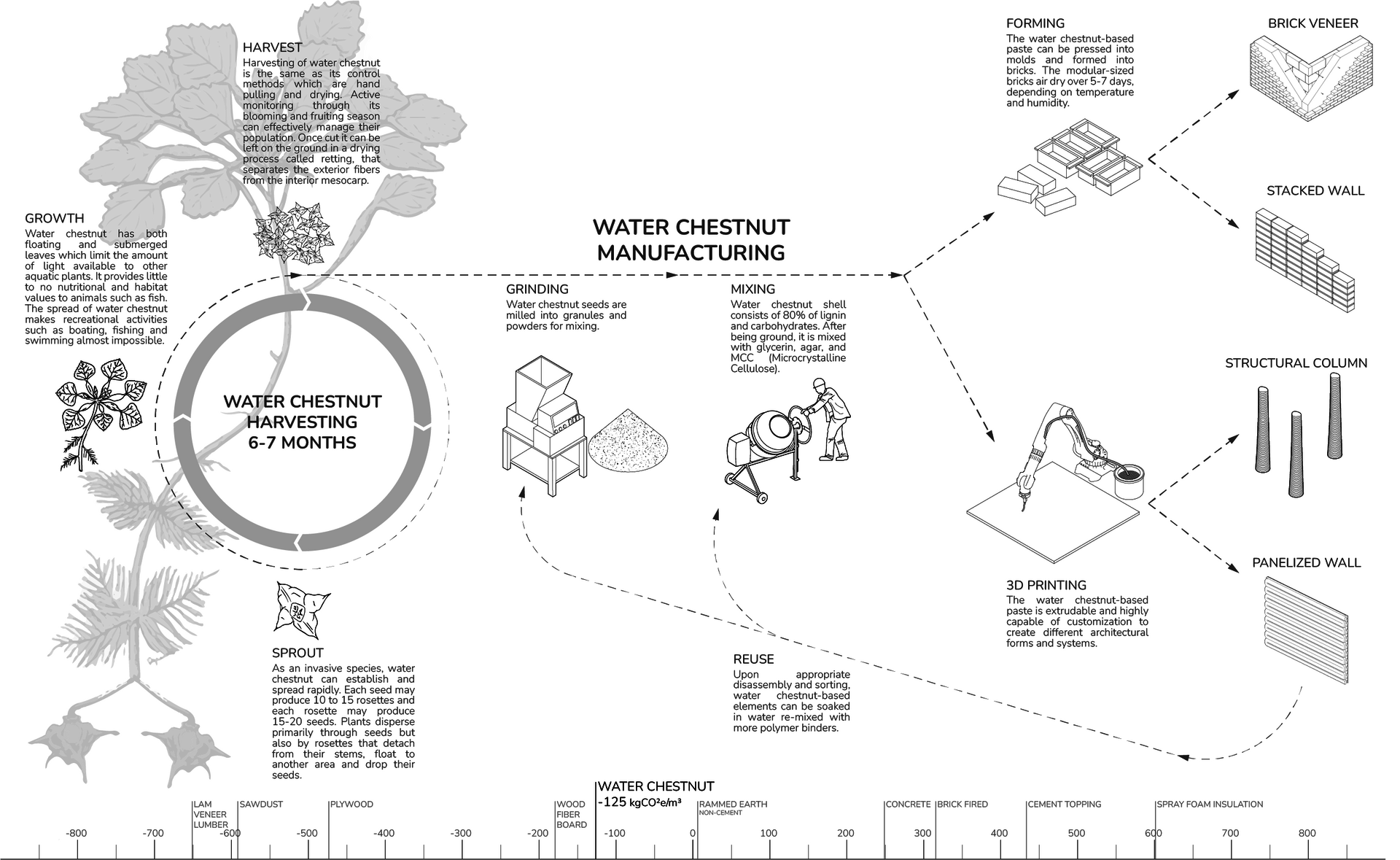
Life Cycle: Water Chestnut Manufacturing
These biogenic materials can inspire designers and architects to reconsider material choices, construction sequences, and tectonic expressions. With their structural potential, insulative nature, high permeability, and excellent sound attenuation performance, they offer versatile and abundant applications. They can be utilized not only in conventional methods like wood framing and masonry but also in thick monolithic structures, capable of multitasking as a building element and offering numerous possibilities.
Image
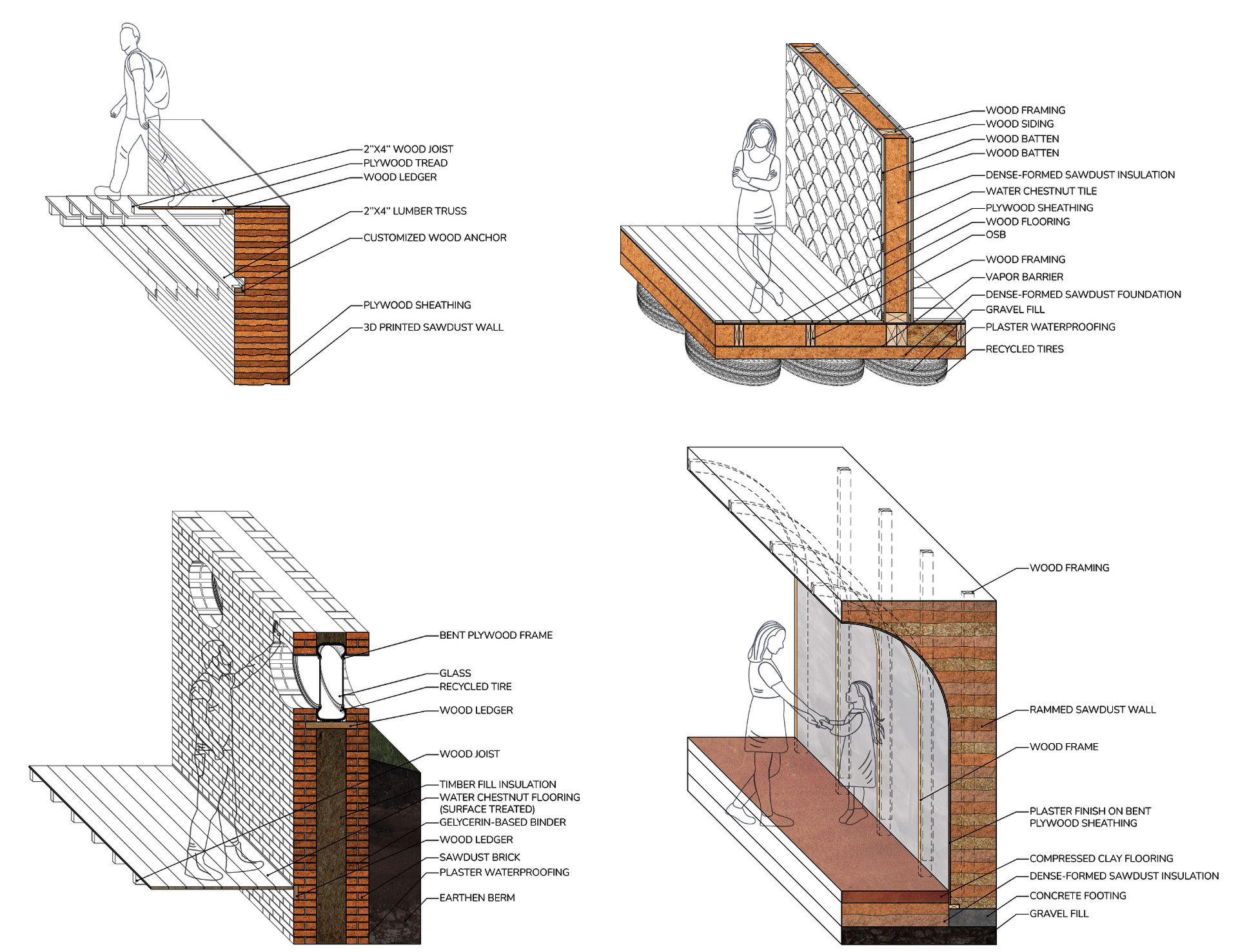
Architectural Systems of Biogenic Materials
The Naushon Mill, abandoned since the 1950s, undergoes transformation into a biomaterial research and manufacturing facility. This thesis advocates for a non-conventional renovation approach, where the primary program (biomaterial 3D print factory) begins simultaneously with the renovation process.
By equipping the sawtooth roof with PV panels to power robotic arms, the renovation can heavily rely on renewable energy and automation. Interior volumes of varying sizes are 3D printed to accommodate different programs. By redefining the thermal envelope within the new interior rooms, the entire facility can function with significantly lower operational carbon emissions and potentially be fully sustained by on-site renewable energy.
The interstitial space between the research lab and workshops is utilized for material drying and panel preparation before transportation via electric trucks.
Image
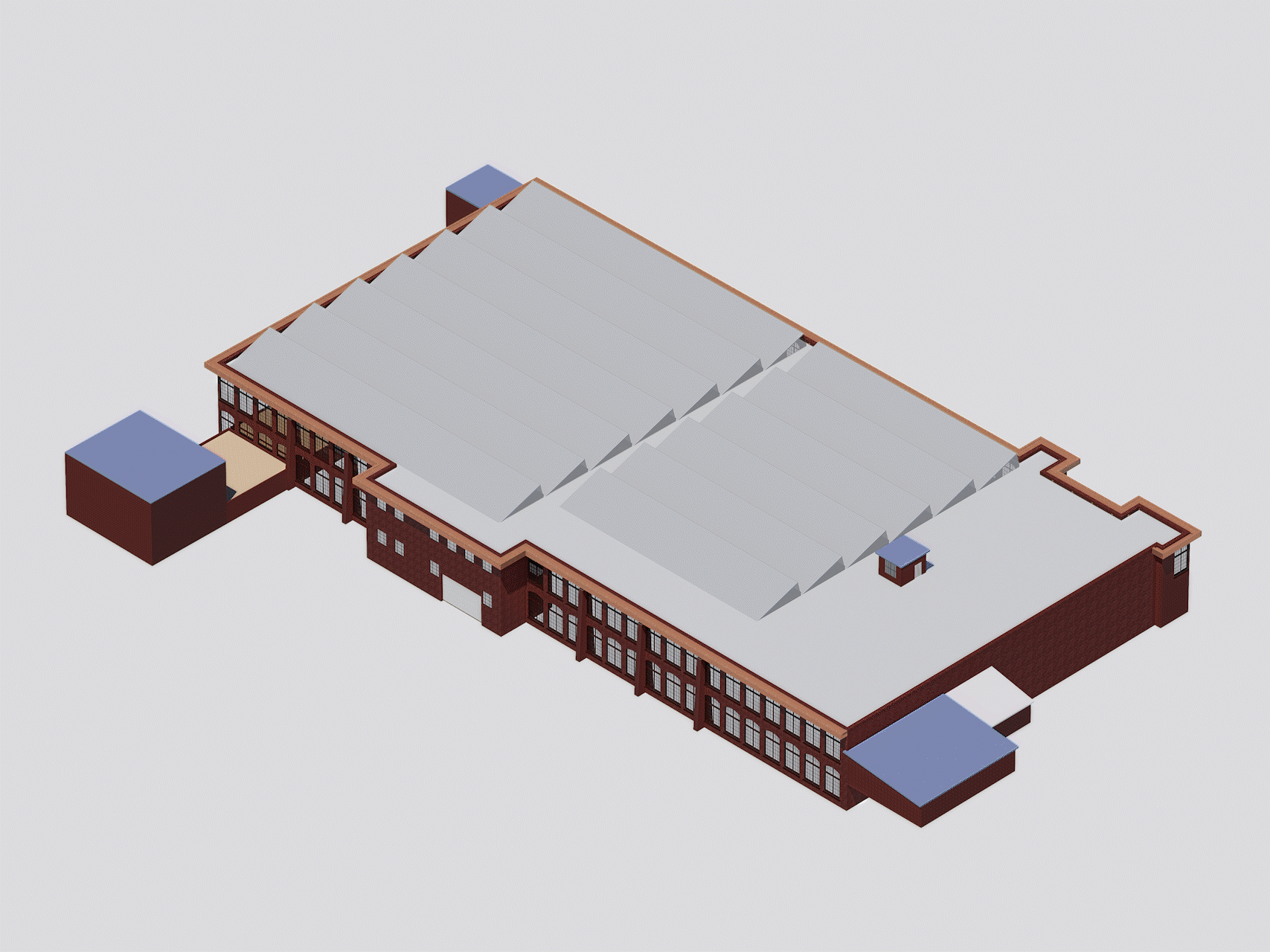
Naushon Mill Adaptive Reuse Animation
EXHIBITION IMAGES